Technology Morphology
Make Me a Bicycle, Clown!
S. G. Lacey
​
Rustenberg, North West Province, South Africa – November 2016
Light flickers eerily, headlamps casting long shadows and bright reflections off the moist, faceted walls of the cave. Once the eyes have adjusted to the inconsistent illumination, smell moves to the forefront of awareness. The aromas in the cave are thick: a combination of damp earth, diesel-powered generators, and the lingering pungent smoke from blast charges. All these flavors are mixed and intensified by the minimal airflow in this claustrophobic passage.
It’s interesting to realize that in a production mine setting like this, sound is one of the least perceived senses. Mandatory hearing protection, combined with the constant hum of machinery that hovers around 95 decibels in the echoey cavern, quickly renders individual noises and verbal communication moot.
Air-driven, water-cooled, hand drills, equipped with lengthy, rattling drill bits. Squat dump trucks on oversized, knobby tires, powered by grumbly engines with no mufflers. A bank of massive compressors providing pneumatic power for the various mining tools, while also hopefully ensuring sufficient airflow in this stagnant chamber 250 meters below the planet’s surface. All these apparatuses conspire to generate a buzz that a rock concert would be happy to emulate.
Surveying the scene, the most noticeable natural features are the bands of metallic ore that streak along the sides of the tunnel. The main seam, at waist level near the cave’s entrance and angling slightly upward, is clearly visible; a 60-centimeter wide swath of very dark grey rock, with obvious metallic luster when the spotty light hits the wall at the proper angle. This valuable resource has been lying in wait for a very long while, but now its time for extraction has come.
The Bushveld Igneous Complex (BIC), formed around 2 billion years ago, is a region where molten rock from the mantle squeezed up to the surface through cracks in the Earth’s crust. This results in an incredibly rich zone of ore deposits ending in “ium”, including platinum, palladium, titanium, vanadium, uranium, and, most notably, chromium. However, at up to 9 kilometers deep in spots, this geology can present some difficult mining challenges.
This small 65 square kilometer region just north of Johannesburg, South Africa contains an estimated 70% of the world’s minable chromite ore, arranged in stratiform deposits caused by large masses of igneous rock which slowly cooled in sub-surface magma chambers. Minerals crystalized first, settling to the bottom of the liquid lava, in clearly identifiable layers based on density and melting point.
A justifiable reason for the decided infrastructure investment at this mine; especially since at current extraction rates, the ore reserves here can support 200 more years of chromite mining.
The mine’s layout is dynamically changing, advancing upwards to downwards in jogged steps based on the snaking grey chromite deposit. Each horizontal chamber runs as long as the natural vein stays in view, and up to 20 meters wide depending on the ore quality. Rock pillars are left throughout the cavern to support the rock above, like natural Roman columns, but much more rugged and sporadically placed.
The low ceilings necessitate specific earthmoving equipment, in this case maneuverable loaders with 2-meter diameter wheels that reach almost as high as the operator’s cab. As soon as a section of the chromite vein is blasted, these slinking haulers move in for the kill, scooping up to 8 tons of rock at a time, then deftly maneuvering backwards to the drop point.
For shallow chromite pockets, the ore can be loaded into 40-ton trucks and driven out to the surface via adits; this layout is very desirable from a cost and efficiency standpoint. At more substantial mining depths, chunks of metallic rock are distributed into small carts, then hoisted vertically to ground level. Once this chromite ore reaches the external processing facility via truck or elevator, it’s milled and loaded onto rail cars for transport.
At the end of this specific tunnel, the lift platform is tiny, nothing more than a 2-meter square cage of rusty, metal bar stock. The rock walls of the shaft have been covered with a thick coating of sprayed concrete, part of the critical extra reinforcement precautions which must been taken around load bearing underground features. This access point also represents the location where key support systems from above: water, air, electricity, are provided by a stout black tube running parallel to the elevator shaft.
Mockingly, a small plastic first aid kit has been velcroid to the girthy service pipe. Once adored with the traditional white and red plumage, this case is now a muted grey color, symmetrical cross on the front barely visible, nearly covered with a thick layer of mining dust, just like everything else around.
Raw chromite mining is just the first step in a long journey of processing, using smelting or roasting and leaching, to generate a useful industrial material. Chromium is a critical alloying component in stainless steels and aluminums, providing both strength and corrosion resistance benefits. Additional uses are as a reflective yellow paint pigment, common on school buses and road center lines, plus for thin, durable, cosmetic chrome plating finish treatments.
Chromite ore is the raw material starting point for all these applications, and the vein in this current chamber has been mined to depletion. Terminating at the ceiling level of about 4 meters, the dark grey chromite seam must be followed upward. To facilitate such efforts, in the top, right corner of this cavern, a narrow shelf has been cut into the rock wall.
The visible chromite line is currently getting blasted with highly pressurized water. The spray is an ancillary result of activity by two middle age workers, both South African locals, who are focusing all their intensity on the 3-centimeter diameter by 1.5-meter long drill in their grasp.
Spreading their legs wide, men and machine operate in unison, 5 hard points of contact on the tiny ledge in the hope of eliminating slippage. The key resisting force, the masonry tip drill turning at 500 revolutions per minute, must be held at the desired angle to track the chromite vein.
5-centimeter-long orthogonal chucks of valuable rock, with 2-millimiter visible grain size, fall to the floor of the cave at the mercy of the miners’ manual drill assault. The primary goal of this probing operation is to provide channels, where small, localized blast charges can then be placed.
The outer facets of the vein are already yielding. As the drill bit hits the desired depth, both the cooling water spray, and pneumatic inflow powering the tool, stop.
Content with their handiwork, the tandem operators extract the spiral rod, and without a moment’s pause, reposition on the next red “X” at the top edge of the chromite vein. The shrill whine of metal on rock fills the mine cavern, another contribution to the endless chorus of heavy machinery.
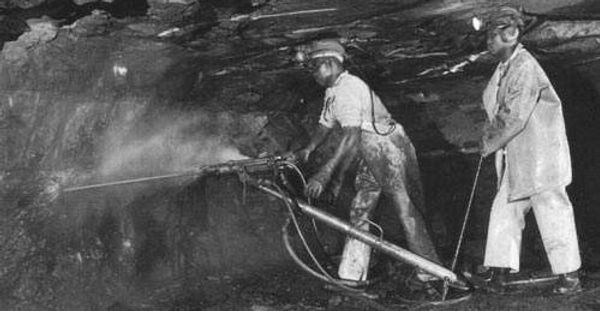
Offshore from Tuban, East Java, Indonesia – May 2017
Relentless waves crash against the side of the hull; 4-meter swells that would strike fear into the heart of many a sailor. However, this 300-deadweight tonnage vessel, when fully loaded, is over 325 meters in length, and therefore essentially indifferent to these thrashing seas.
Ship may not be an accurate description, since the heaping hunk of metal is now a permeant fixture at this location on the ocean, having not moved off its moorings in 3 years. This massive floating storage reservoir, with a capacity to hold 2 million barrels of crude oil at one time, is part of a Floating Storage and Offloading (FSO) site.
The facility is aptly named after a midnight-colored stallion in mythical Indonesian lore, as today it provides a continuous, powerful flow of black oil to the masses.
Sitting behind the FSO complex is a smaller tanker, smaller being relative since this boat still has a deck over two and a half football fields long. A robust, yet flexible, hose currently connects the two hulls, with crude oil flowing out of the storage vessel and into the tanker. With a carrying capacity of over half a million barrels of its own, the pending exchange will take a few minutes.
Bobbing between the two large boats like a rubber ducky in a frothy children’s bubble bath, a small diesel-powered ship maneuvers to and fro, sailors rigging ropes, maneuvering chains, and connecting hoses. Though dwarfed by the two larger vessels hulking above, this line handling skiff is tasked with the key role of maintaining the tight spacing required for the oil transfer process.
When connected, this floating chain stretches out to nearly a kilometer, with the rolling seas presenting an additional burden to the already challenging logistics. A little more complicated than a quick gas tank fill-up at the local service station.
The main reason for the current congregation of ships at this particular location is the FSO tower yoke. An odd structure, this metal frame in the middle of the ocean, 23 kilometers off shore, resembles a bridge pylon which engineers forgot to attached the roadway to. The pile-driven structural elements occupy a surprisingly small square footprint just 40 meters to a side. Water here is relatively shallow, less than 25 meters, which simplifies ocean floor construction, while still being deep enough to accommodate the wide variety of tankers that frequently visit the site.
The more relevant geometric feature is the base platform, which is round as opposed to orthogonal, and mounted on bearings to allow the entire upper portion to rotate through a full 360° arc, as dictated by wind and ocean currents.
A broad range of tubing diameters are utilized on the structure, as if the welders haphazardly tacked on whichever pipe size was convenient. However, the engineering is precise, with total structure mass being reduced as much as possible to optimize swing weight. A wise decision, considering the triangular yoke appendage, which is cantilevered well off the water, serves as a connection harness to the massive floating, flammable container it feeds.
The FSO tower is supplied by a pipeline which extends from the cold blue ocean water below, up through the center of the structure, then out across the mooring yoke. Ancillary cables for power and hydraulics sag above this rigid frame, allowing flow control coordination between the pipeline tower and floating storage vessel.
The sea is a harsh place for machinery. Sun bakes the exposed metal surfaces. Powerful waves blast the support structure. The salty operating environment perpetually corrodes from below. As a result, maintenance is frequent, and costly.
The petroleum currently at this FSO site has traveled almost 100 km from its extraction in the Banyu Urip oil fields, part of the Cepu Block, which straddles the border of Central and East Java in Indonesia. The land-based Banyu Urip central processing facility refines and treats the raw crude before sending it along to the FSO, through a permanent pipeline half a meter in diameter. Rapid liquid flow rates allow for throughput above 200,000 barrels per day at peak production.
Most of the petroleum pulled from the Cepu Block region, which is estimated to have over 700 million barrels of accessible reserves, is destined for domestic distribution within Indonesia, per government regulations.
However, the tanker currently being filled has a different destination, northward to Japan, and atypical product end state, butyl rubber. This highly impermeable material is commonly used for safety gloves, and tire bladders.
The oil extracted from the Cepu Block is medium-heavy sweet crude with low sulfur content, making it valuable for a wide range of applications. Through a variety of absorption and distillation refining techniques, the raw hydrocarbons can be used to obtain the isobutane required as the input for making butyl rubber.
Filling complete, the surprisingly still-seaworthy tanker ship disconnects, and chugs off. With a max speed of 20 knots, stopping distance of 6 kilometers, and turning radius of over a kilometer, this is not an agile vessel.
Piracy has become an increasing concern for these large, sluggish oil tankers, especially around the Strait of Malacca, a key logistical route between the Indian and Pacific Oceans. Despite these risks, due to the high demand for oil in Asia countries like Japan and China, 15 million barrels pass through this shipping bottleneck just south of Singapore daily.
This tanker transfer and distribution is just an intermediate step in the long and complex hydrocarbon journey, starting with a 100-million-year-old fossil fuel being extracted from the ground, and finishing with a petroleum rubber used for commercial product applications.

Bay Area, California, USA – November 2017
This is the largest conference room available at corporate headquarters, and it’s still crowded. Stuffy is an accurate descriptor. The stale air mingles with the aroma of sweaty bodies, and the remnants of takeout Chinese food catered lunch. The weather outside is not so much cold as damp, typical for San Francisco this time of year, and a stark contrast to the steamy atmosphere of this inner war room.
A PowerPoint projection graces the entire front wall, flanking whiteboards are covered with scrawl, and the wooden boardroom table is littered with printouts. Cups of coffee, some recently filled, some empty for hours, dot flat surfaces throughout the battlefield. An easel of chart paper in the corner is devoted entirely to acronyms: TLD, ITL, PLM, BOM, each with a multitude of numbers that appear to be dates listed next to each cryptic term.
Though no actual design specifications will be set at this meeting, the brainpower and knowledge in the room represents the collective which will shape the direction of the product line moving forward. This meeting is a biannual occurrence, defining not only the high-end models, but also the takedown plan that will scale to lower price point offerings.
Marketing, product, design, engineering, supply chain, sales; these teams all get their time to present opportunities and concerns. Key variables to balance include projected consumer trends in the industry, current merchandise sell-though, new performance technologies, corporate growth strategy, and a multitude of other smaller considerations.
Not one, but two secretaries are taking notes, and the entire meeting is being recorded on video to document the decision-making process. Red Sharpie diagram lines, yellow highlighted text, orange Post-It notes; the colorful trail of proposals and conclusions is impossible to keep up with.
Many of the assembled participants have adjusted holiday travel plans to attend this product line kick-off, some traveling into town from Europe and Asia. Now, at past 4 PM, as the meeting drags on, many individuals start mentally checking out for their Thanksgiving holiday break.
3 hours later when the meeting finally adjourns, the vibe is a mix of excitement, frustration, and anxiety. The 2020 model offerings are set. Now, all that needs to happen is almost 2 years of solid corporate product execution.

Taichung City, Taiwan – January 2018
Mice click and computers whir. 3D images move freely on the workstation monitors, shifting in and out of the page, twisting around incomprehensively, while toggling rapidly between views. This digital dance is not as much aesthetic as it is functional.
12 high-powered CAD work stations are positioned in a semicircular ring, with partitions between each operator’s desk to avoid the inevitable distraction of the jittering adjacent screen images. But make no mistake, this team is as collaborative, and connected, as any in the sport equipment engineering space.
Most CAD technicians wear headphones, listening to a variety of music, depending on which style helps each operator focus on the task at hand. Their language of communication is visual, not verbal, with 3D models for various components being constantly updated and saved on the shared server.
This method allows assemblies to be made by the collective group, redundantly confirming fits and tolerances. Every operator has their own area of expertise, be it 3D surfacing, dimensioned technical drawings, assembly diagrams, or mold design.
In the middle of this half circle of desks is the keystone that unites and supports these modelling efforts, not virtually, but physically. A fully assembled mountain bike, with tiny, laser inscribed, 5-digit part ID numbers attached to each individual component. These codes tie back to an online database with more detailed part name information. On some models with more innovative design features there may be a multitude of unique, new parts, but over 80% of mountain bikes utilize the same basic shared componentry: derailleurs, cranks, brake levers, headsets, brake calipers, etc..
The adjacent room houses two of the most prize processions for this constantly revolving engineering exercise. In one corner sits an 3D printer for nylon 12, complete with all the necessary accoutrements like a sandblasting box and material recycling bin. Everything is perfect in the virtual world, but in real life production tooling is expensive. Better to rapid prototype components to confirm the surfacing, dimensions, and functionality are acceptable before spending $20k on a plastic injection mold or metal forging tool.
The 3D scanner, and accompanying CMM arm mounted on a precision marble slab table, are poetically placed in the opposite corner of the cozy room. While the FDM rapid prototyping transfers virtual CAD models into reality, the scanner verifies that reality is what it claims to be, and even more importantly, transfers physical parts back into the digital space with brutal accuracy.
Every parting line, injection gate, mold finish, and shape distortion become evident under the watchful eyes of the scanner, augmented by dimensional inspection. Granted, the process is time consuming, requiring several hours, and multiple fixture locations, to get a full 3D part model, since the various views need to be spliced together in CAD. However, when done correctly, the truth doesn’t lie in a precise physical world.
This particular project has a compelling sense of urgency, with the goal being to release the new CAD models, and assorted fully dimensioned drawings, by the end of the month. Such timing will allow tooling development before the upcoming Asian Lunar New Year in early February.
Armed with this powerful collection of machinery, the aggressive timeline might just be feasible. Computers render, 3D printers hum, scanners resolve, and functional prototype mountain bike drawings are created en masse, and hopefully on schedule.
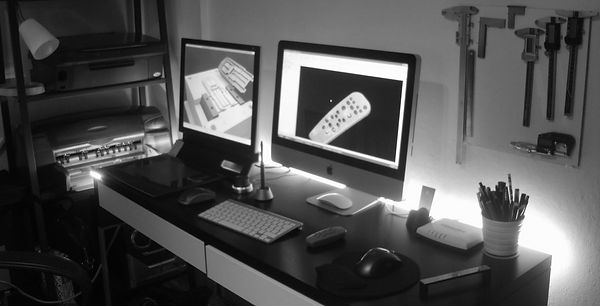
Whistler, British Columbia, Canada – May 2018
8 identical, matte black mountain bikes, with no corporate branding or graphics of any kind, sit in wait on the rack. The two missing slots are a tell-tale sign of current activities. 2.5 kilometers away, and 300 meters above this location, these absent vehicles are barreling down the slope at rapid speed.
This fact is easily verified remotely by the laptop sitting on a table under the pop-up tent, shielding its occupants from the late afternoon sunlight which could potentially cast a glare on the monitors. Beyond simple tracking data like GPS location in 3 dimensions, the sensor systems on these bikes can track shock loads, speed rate of change, and vibration frequencies, all via accelerometers placed on both the bike and rider.
Sure, heart rate, O2 utilization, and other human metrics would be fun to track, but when you’re using the best athletes in the world for product testing, the physical element becomes negligible relative to even minute changes in product performance.
The snow on most of the mountain has finally thawed, and skiers are now turning into mountain bikers, who can’t wait to get back in the saddle. Current testing is being conducted on the flowy, lower trails; evaluation of prototype mountain bike performance on the upper runs, with their exposed rocks and steeper sections, will need to wait until later in the spring.
Adjacent to the analytics tent, the traveling bike service truck is humming with activity. Each rider and stead must be instrumented, robustly enough to handle the 7-minute test loop, with small sensors which will go unnoticed by operator or machine, as such incumbrance could influence the outcome of the testing.
First test lap complete, two incredibly muddy bikes return to the staging area, and two fresh machines leave the rack. Same riders, same course, different machinery. Granted, they have the 5-minute mid-station gondola ride to recover, but the toils of an A-Line descent lingers for days on most people. These athletic adrenaline junkies are not most people.
Meanwhile, the next set of test subjects has arrived; two women with MTB pedigree and experience. A very important data set.
It’s critical to evaluate multiple frame sizes. Weight, stature, and strength all play a role in the balance between stiffness and compliance on a full-suspension mountain bike system. You can meet a guy at any random bar on a Tuesday in Whistler who will brag about his epic mountain bike adventures, many with solid justification, but hard riding ladies are more difficult to find.
By 7:45 PM, the light, and more importantly batteries on all data collection devices, are fading. There are hours of spreadsheet numbers to analyze, plus the high-speed video captured by an ambitious intern at one of the key turns on the descent around the 900-meter elevation level. Hopefully she makes it back to the village safely before the daylight completely gives out.
Time to meet at the bars for a beer, the testing crew has earned it, and the riders will be there anyways. Another good day at the office.
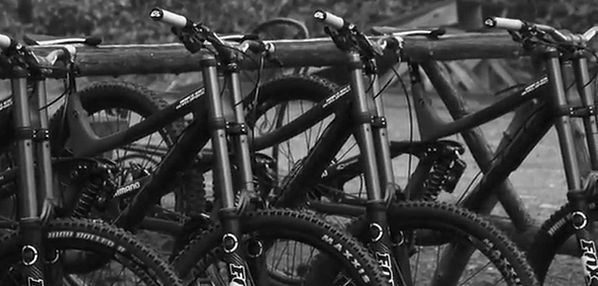
Osaka, Honshu, Japan – September 2018
The facility’s organization is immaculate in true Kanban fashion. The surgeon-like procedures executed here are timely, precise, and efficient. This could be confused for a hospital, except that several of the machines are more expensive than a sophisticated MRI scanner.
Observing from the catwalk above the factory floor offers a bird-eye view into the manufacturing process and progression of metalwork steps. In an isolated, climate-controlled room off this raised platform sits a bank of computer screens. Closely watched displays report key production throughput metrics for the entire shop floor below in real time. Every machine is monitored, every part tracked, every environmental condition set. Nothing is left to chance in this manufacturing facility.
The singular goal of this sprawling work cell is to take large, thin sheets of stainless steel, and turn them into precise, functioning gear components. The required series of procedures is well-known, but complex: water jet blasting, precision hole drilling, shaping of the critical teeth geometries, final curing with heat and time. Though using similar terminology, the metalworking activities occurring here are not the best analog for dentistry either.
Metal arrives by truck in flat sheets, already having been precision rolled to the desired thickness of just under 2 millimeters. The water jet cutting machine can accommodate full panels up to 3 meters in length by 1.5 meters wide. A hydraulic crane with suction cup arms lifts these heavy slabs, and swings them over into place on the water jet bed, thus eliminating the need for human exertion.
The cutting path for the entire sheet has already been programmed into the computer controllers. These round parts are nested as closely as possible to eliminate waste, using a predetermined reference location on the near corner of the orthogonal sheet for an origin coordinate reference point.
As the water jet hums to life, pressure at the nozzle exceeding 500 megapascals, the protective plexiglass cage around the table becomes awash with a frothy spray of water mixed with the fine garnet abrasives used to aid in the cutting process. The base sheet stock immediately becomes obscured from view, but the CAM controls of the jet head ensures precise geometries are achieved part after part.
2 hours later, a single stainless steel sheet has been converted into 502 individual gear blanks, along with a complex piece of waste that resembles a geometrically-obsessed spider’s web. These sheet scrap negatives are retained for recycling, while the desirable components get tumble sorted into bins by size, and move on to the next step of their journey via conveyor belt.
Though the parts at this stage are already slightly suggestive of a bike sprocket, the precision manufacturing is just beginning. Rough shape established, the stainless steel blanks are next modified using progressive forging to achieve the desired cog tooth profile. This process uses extensive tooling, and requires massive amounts of pressing tonnage, but again is virtually free from human labor input.
Cold forming has the benefit of not manipulating the grain structure of the metal substantially, since geometry shifts happen slowly and in a controlled manner. This is important when the target final product, an 11-gear cassette assembly in this case, requires precise tapered tooth profiles just 0.2 millimeter at their edges, with a 5-micrometer tolerance. That level of exactness makes the center of the sprocket, at 1.5 millimeters thick, relatively easy to control.
Each forming step requires a different mold, made from hardened tool steel, which will not deform under the massive pressing loads incurred piece after piece. Cold forging is done at room temperature, with the part moved sequentially down the pressing line automatically, and incrementally shaped until the final cog geometry is generated.
The nuances identifying different models of sprockets are indistinguishable to the naked eye. Unique steel alloys, varying gear diameters, minute changes in tooth tip profile; all these elements need to be tracked during the manufacturing process. As a result, parts are closely monitored and labeled using a sophisticated laser-marking bar code system, to ensure the correct assembly protocols for each cassette model.
The final step of the bicycle sprocket creation process is to heat treat each component. Depending on gear diameter steps on the cassette, only 3 to 5 links of chain are engaged on the cog teeth at any given time during shifting. This can result in tremendous forces at the tip of the tooth, as a rider drives down hard on the pedal during a grueling hill climb. Hence, the necessity to optimize the mechanical properties of the final stainless steel product.
Done in a specific series of steps depending on the alloying constituents, the part is annealed at medium temperature to unify the grain structured, treated at a precise higher oven setting to promote the desired microstructure, rapidly cooled in water to quench and lock in the atomic orientation, then tempered as needed to reach final desired hardness. Key parameters like time and temperature for each thermocycling step are close monitored from the control room above.
The team here in Japan didn’t get their expertise in metalwork overnight, in fact it took approximately 15 centuries, not years. The Japanese tradition of steel craftsmanship, starting with ploughs for farming, then transitioning into weaponry, Samurai swords, cutlery knives, and gun barrels, for military applications, and now bicycles, is a complex and twisted history, though the basic metallurgy principles have remained essentially the same.
Sure, the tricks of the trade have changed slightly, as evidenced by the robotic forklifts, CNC cutting machinery, high-tonnage hydraulic presses, and ovens with electronic temperature control, but the dedication to quality metal component craftsmanship has not.
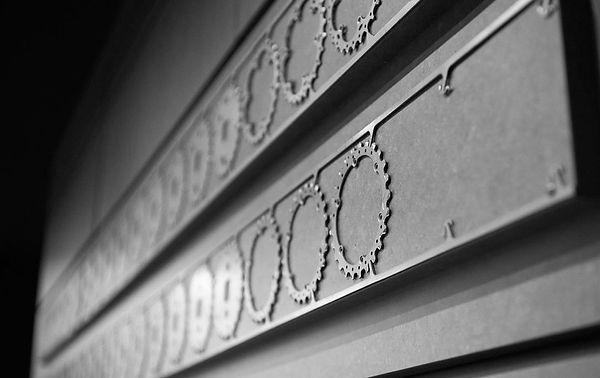
Givisiez, Fribourg, Switzerland – January 2019
A black cloth shrouds a 2-meter wide x 1-meter deep folding table, likely stolen from the cafeteria downstairs. The back and sides of this raised surface are ringed by black foam core, slit halfway through on the corners to create a 3-sided pen. A second cloth of the same dark color and composition is stretched tightly across the top of the board and duct-taped into place on the back side.
Two cone-shaped fixtures on tripods cast powerful white florescent light into this makeshift cave, while multiple tiny articulating LED bulbs sit on the table, their power cords dangling down ready to be positioned into place at a moment’s notice as needed.
Inside this enclosure sits a gunmetal grey bike frame; pinstripe accents in gold metallic and rich black, with primarily white logo branding. However, these elements stand out much differently than traditional decal-on-paint highlights, as they are glossy, providing a stark textural contrast to the tonal matte grey which dominates the palate.
There are approximately 15 people in the room, most huddled around the spot-lit frame, while an animated woman of approximately 40 explains the various design elements in a broken combination of English and Italian.
Her eyes flit left and right, hands leading the engaged observers to various parts of the cosmetic treatment: the gold is actually aluminum anodizing, which is masked over before the matte clearcoat application. Same with the black regions, which actually reveal a 2 mm square woven carbon fiber twill pattern upon closer inspection. Subtle raised lines between the gloss and matte clearcoats, perceptible to the touch, give away the secret to this cosmetic execution, but nobody appears to mind.
Seemingly satisfied with the first part of the demonstration, she signals for the lights to go out, but the colors of the logo do not disappear. Instead, they simply shift from bright white to an eerie dull green, like a kiwi blended into a glass of milk. The observers nod, cooing with content as a cat would when petted appropriately in an owner’s lap. Emboldened by this reaction, the presenter proceeds like a magician in mid-act, gaining confidence with each successful new reveal.
And now for the final act. Grabbing a modern Polaroid camera from her box of goodies, the leader of this charade snaps two pictures in quick succession, one side-on of the primary branding logo on the downtube, and one of headset, as front-on profile as possible without disturbing the foam core wall at that end of the enclosure. The onlookers blink reflexively, the bright light of the flash is blinding in the still darkened room.
Waiting the obligatory 15 seconds, the printed pictures, no negative exposure needed, come into focus, and are passed around. The result is compelling, two brilliantly highlighted, stark white, logos against the grey-black of the remaining painted frame, silhouetted by the even darker background behind. Apparently, next time a world-class mountain biker goes for a nighttime hairline decent, they’ll be all set for a magazine cover shot.
3 hours later, after much deliberation, mostly focusing on the high-resolution color renderings that have been printed out in full scale, and pinned on whiteboards surrounding the makeshift stage, the final 2020 colorways are selected.
No surprise, the team is proceeding with red and green on the men’s side, and purple for the ladies; all 3 hues are currently trending in consumer products, and more importantly, common aluminum anodizing treatments, which helps with finishing execution.
The security sticker is a go, the hologram approach preferred over UV light activation, along with the matte-on-gloss composite window treatments, which are almost mandatory in this age of compelling cosmetics that must highlight the underlying technology. The team decides to hold off on the glow-in-the-dark and reflective decals until the company starts selling headlamps.
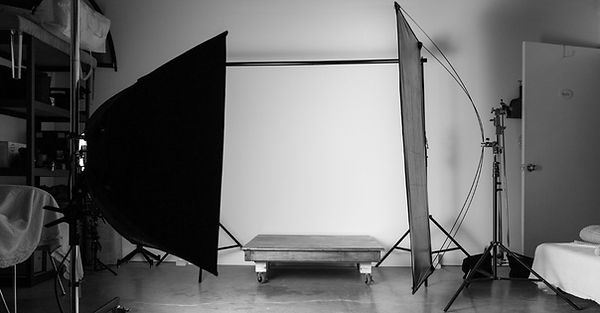
Verkhnaya Salda, Sverdlovsk Region, Russia – February 2019
The racks lining both walls contain immense amounts of metal bar and rod stock, both solid and hollow. It’s impossible to estimate how many cross-sectional geometries are represented, even before accounting for the different metal alloys each length might be made from. Whoever handles inventory management in this facility must have a full-time job.
Amusingly, these racks appear to be made with some of the very extruded stock that they are holding. Like the metalworks’ inventory, the rack’s construction is haphazard: some bolted, some welded, some with threaded mating elbow fittings. The only constant is that all shelves are bowing under the weight of their heavy burden.
Despite the brisk outside temperatures, the factory floor is a combination of stifling heat, moist steam, and pungent cigarette smoke. The source of all three atmospheric contributions are readily available by examining the ongoing activities.
This building is not as much expansive as it is lengthy, only 20 meters wide, but over 100 meters long, open down the entire center with the eternal walls providing the necessary roof support.
Along one main side sit round plugs of metal, much more consistent in diameter and length than the tubular products they will become. Denoting of alloy uses the commercially accepted, but seemingly archaic, system of colored spray paint on the top of each ingot. Blue dots, red dots, black dots; nearly every common aluminum grade is represented.
These billets are stacked like logs in a wood pile, which they resemble very closely in size. Each pyramid arrangement is constrained on a standard wooden pallet, supplemented with low corrugated metal sidewalls, which keep the entire precarious stack from rolling away. An operator on a Cold War era forklift picks up a triangular tower and transports it to the back end of the room, puffing away on a cigarette as he goes. One atmospheric anomaly identified.
In the center of the warehouse sits the facility’s key piece of machinery. A hulking unit which resembles a scaled-down version of a Space Race era rocket, complete with boosters, but tipped on its side as opposed to standing upright. The 4 horizontal structural members in the corners hold the machine together, and oil seeps from multiple loose hydraulic fluid connections.
At the business end of the machine, a 30-centimeter diameter by 15-centimeter thick hardened steel die sits in the center of the structure. The precision aperture machined into this die represents the final shape of the pending extrusion.
The digital central control panel for this extrusion machine is space station MIR-like; with no less than 25 buttons of various sizes, 3 analogue time/temperature displays, plus a supplemental black and green screen that a DOS computer would be embarrassed by.
The forklift driver’s cargo of round billets is now being rolled onto a V-shaped conveyor belt at the back end of the press. These silver logs chug slowly forward towards their destiny, passing through a gas-powered furnace, which gets the aluminum to a temperature just north of 450 degrees Celsius. Nothing about the process from here on out is cold; which explains the incredible heat this massive extrusion apparatus is throwing off, effectively warming the entire warehouse space.
Exiting the oven, each subsequent tube is picked up by a rotating mechanical arm and positioned at the machine’s entry aperture. With impressive timing and efficiency, as the new billet is rotated into place, the remnants from the last extrusion cycle are cleaved off the press ram, which then moves forward into position against a new foe.
Initial engagement is rapid, the now joined billet and ram sliding quickly forward towards the die opening. Once resistance is met, the hydraulics adjust, ramping up to a pressure of 500 megapascals, which forces the softened, but still integrally structural, aluminum through the much harder, and more temperature resistant, tool steel die.
It takes about 5 seconds to push the entire meter long aluminum billet, over 70 kilograms, through the die, but the truly impressive activity occurs at the back side of the tool. Barreling out of the die at 12 meters per second, is a thin-walled tube of aluminum with a 27.2-millimeter outer diameter, mimicking the geometry of the opening it has just passed through.
Just after exiting the extrusion phase, this newly created bar stock passes through a cold-water bath, exciting a vigorous thermal reaction and producing, yep, steam. Lots and lots of steam, which eventually settles and condenses, droplets collecting and rusting any metal that is not aluminum or stainless steel in the facility. This includes the corrugated roof, worn hand tools, exposed electrical wiring, and pretty much every other surface in this outdated facility.
Straightening, cutting, and heat treating occurs downstream, but this monstrous machine is where the magic happens. With aluminum extrusions exiting the die faster than the average human can run, it makes sense to have a facility the same length as a Soviet nuclear submarine.
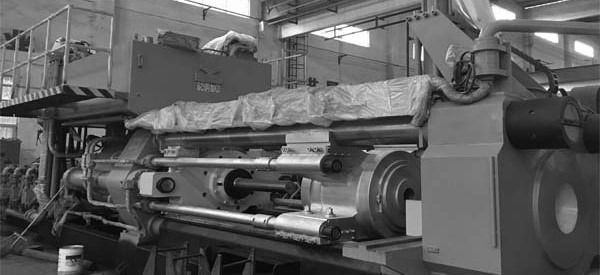
Xiamen, Fujian, China – March 2019
This is not a typical university laboratory setting. While the participants are all wearing white long-sleeve coats, blue latex gloves, and recycled brown-paper filter ventilation masks, there’s no biology research going on here. This is a full-on production setting, each step of the manufacturing process designed for optimizing quality, cost, and, most importantly, efficiency.
Rather than tracking an operators’ movements, 80% of which are women, the easiest way to understand the flow of fabrication is to follow the material itself. Rooms are separated and logically numbered, to segregate different procedures and workers, but also to provide a spring-like buffer in case one link in the industrial chain gets snagged, or races ahead.
Here, behind a door labelled Room #3, one of the more important steps in the composite manufacturing process happens. Operators sit at low metal stools, in front of battered wooden tables, covered with smooth Teflon sheets. On the shelf above, at about eye level and just within arm’s reach, are plastic bins with reference #’s on them, and different shaped pre-preg pieces inside. Next to every worker is a detailed paper schedule which identifies the order in which each layer should be assembled to create the final preform for molding.
Down the hall, in Room #1, the base pre-preg formulation, a key raw material input for composite manufacturing, is created. Here, carbon fiber tows are unfurled off 10-kilogram rolls, over 6000 meters in length, and pass through a liquid matrix dipping bath onto non-stick paper.
This is the most boring of the pending composite processes, but arguably the most critical. These pre-preg sheets being rolled onto large drums are the feed stock for all the downstream activities. Efficient control of the fiber angle and carbon-to-epoxy content for this input material ensures the desired product performance.
Finished sheets of pre-preg are cut off the drums, then transported onto metal wire racks, each allowed enough room to avoid sticking to its colleagues above and below. These plywood-sized unidirectional fabrics are nearly identical tackiness and consistency to fruit leather. Also, similar to candy or gum, once the non-stick packaging paper is removed, handling becomes much more challenging.
As a result, in Room #2, the various pieces required to create the specific composite hand lay-up are die cut out using simple presses, leaving the paper on. Here, extreme care is taken to monitor the fiber orientation for each swatch. The optimal nesting pattern is already calculated, operators simply rotate the sheet appropriately, then stamp out pieces with robotic speed and precision.
These initial upstream processing steps are simply the precursor to the actual composite product execution that happens here in Room #3.
This composite kitting process seems easy, like a jigsaw puzzle or color by number painting. However, each pre-cut ply is almost identical in color, fiber direction nearly indistinguishable, and many of the swatches have mirrored shapes. Plus, the operator must place 30 pieces per minute in the correct orientation to complete their task, and that’s just for the bike’s top tube.
13 minutes on a running per part clock, and 8 workers later, the composite lay-up has been completed. Another complication is that for hollow parts like bike frames, a bladder must be inserted for inflation during molding, thus the necessary pre-preg pieces have to be laid up around this sacrificial air tube.
Looking further down the hallway, Rooms #4 and #5 contain operations for molding and trimming of the composite part, identical white doors equally spaced and sequentially numbers. This is the least traditional laboratory environment around, but, just like advanced scientific research at a university, process controls and quality inspections are critical, to final composite part performance.

Hartmannsdorf, Saxony, Germany – June 2019
The assembly line philosophy hasn’t changed much since the days of Henry Ford’s Model T, and surprisingly the original lingo still accurately describes the basic manufacturing scheme. Sure, assembly cells have gotten more compartmentalized, and nuanced, with improvements in automation. But generally, factories have maintained the originally conceived, linear, systematic approach for building complex products.
In this case, the 10,000 square meter production assembly facility is supported by a warehouse space two and a half times larger, housing both required upstream parts, and finished bicycles. At the critical confluence of this layout, a series of sequential tasks intakes the wide variety of available individual components, and spits out a completed, functional ride. Leveraging the factory’s Eastern Germany location, most bikes are fully assembled in this building, then safely protected with padding, and shipped via truck to be sold on the display floor at Eurozone retail shops.
Inventory management of both incoming parts and finished product is critical for maintaining throughput, and hitting the facility’s performance metrics. Forklifts whiz by in various directions along narrow paths marked with yellow; from above this resembles a convoluted, life-size, Pac Mac game.
There are sub-assembly stations for many operations, combining individual pieces into a single unit, which can be tacked on to the bicycle build as it moves down the line.
On cue, a rim is furnished with internal cotton liner tape, while being locked into a horizontal fixture simulating the hub. In quick succession, a rubber tire is seated in place around the rim, then a tube stretched into place between the rim and tire. A third consecutive deft circular motion by the wheel assembly expert seals the internal bead of the tire underneath the top side of the rim. Simultaneously, the tube is filled by an air connection at the tiny Presta stem, which is identically placed on every part via the jig’s design.
The entire process takes 24 seconds; a Tour De France support car team would be jealous, and this employee does well over 1,000 bike wheels per day.
Throughout the facility, the integration of automated and human activities is nearly seamless. The entire building has a linked cadence, like an army moving in unison to the methodical beat of a drum corps. The key large sub-assembly inputs: frames, wheel sets, handle bars, and forks, are carried by conveyor hooks above. No space is wasted, and the timing of each component along the moving belts ensures that productivity is optimally maintained.
White cloth gloves are mandatory for workers at the late stages in the bike’s construction, with the finished product potentially just hours away from a retail storefront, where it will be endlessly scrutinized by consumers.
Also, protective packing in the form of soft foam, plastic bubble wrap, and easy-to-remove adhesive identification stickers have been added to any region that does not need to be accessed during the final assembly processes. This prepares the bike for shipment, while also minimizing damage throughout the final steps of each bicycle’s construction.
In the foreground, a skilled operator executes one of the most challenging operations of the assembly, routing and landing of the brake and shift cables. This activity must be one of the last steps before final functional checks, since these twisted metal wires wind from the extreme forward handlebar levers to key locations on the derailleurs and brake calipers in the rear portion of the bike. The tension on each of these cables in critical to the bike’s function, with the worst-case scenario being a brake pad that doesn’t engage, or a cassette with only one functional chain ring.
As the finished bikes chug steadily along on their path toward final inventory and distribution, inspectors dart in and out of the metallic parade checking various metrics. Most of these are visual cosmetic inspections yet to be automated; performance details like total weight, assembled nut tension, and wheel friction, can be monitored automatically, without human intervention.
Precise and efficient craftsmanship, one 2-wheeled transportation vehicle at a time.
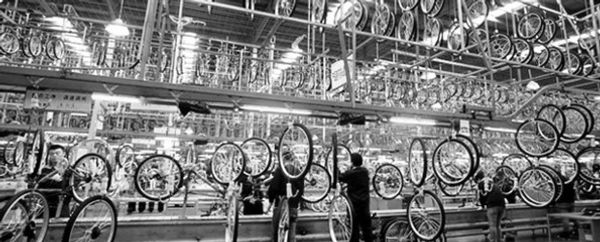
Cordillera del Paine, Torres de Paine, Chile – October 2019
The mountain bike tracks along the ridge trail, a path just 15 centimeters wide that falls away to jagged rock on both sides. The fading afternoon light casts long shadows behind the bike and its operator. The angled slopes skew the image into a grotesque black monster trailing behind the actual machine and its mount.
However, the frame lines and colorway clearly identify this ride as the brand new, high-end, mountain bike model that has just been launched globally. While winter is about to set in throughout the Northern Hemisphere, in this remote southern destination, biking is just getting fired up for the spring.
The rider stands, bending knees and waist as needed to absorb the undulations of the trail. The bike moves in unison, both front and rear shocks flexing smoothly. Knobby 29” tires inflated using air-filled butyl rubber tubes provide a second layer of compliance, absorbing subtle changes in trail surface from a stray pebble or encroaching root.
Aside from the crunch of gravel under the rapidly rolling wheels, and the whoosh of the wind through the rider’s helmet, there are no sounds. Gears shift smoothly, disc brakes engage in silence; both a testament to precision assembly methods. A calm serenity briefly engulfs the picturesque mountain scene.
Suddenly, as the operator approaches a hairpin left turn, it becomes evident that the current speed does not match the tight radius and steep pitch of the upcoming bend. Hitting the brakes too late, the initially true rear tire skids right on the loose dirt, and the bike teeters 45 degrees off its normal vertical orientation for several seconds before conceding to gravity. This is not the functional layout or loading case the CAD designer’s envisioned.
The bicycle and rider, still attached by one foot and one hand, careen off the outside of the narrow track. Fortunately, what lies beyond is not the razor-sharp rocks, which were present trailside just minutes earlier, but instead lush green moss, and in this case an inconveniently placed scrubby cypress bush.
Impact is inevitable, as is an increase in ambient noise. Loose stones rattle down the adjacent scree slope, branches crack sharply as bike and tree connect at high speed, with both natural sounds quickly overshadowed by the audible cursing of this machine’s occupant.
It’s a struggle to disentangling from the fallen bike, which now sports a large gash in the textured matte paint of the top tube, cutting through the bike’s reflective graphics. Fortunately, no scratches have pierced the brittle, but structural important, carbon frame which lies beneath the bike’s flashy aesthetic coating.
The key functional workings of the bike appear intact. The teeth on the largest ring of the chrome-plated, stainless steel cassette are unblemished, even after striking a large, protruding rock during the unintended dismount. Gently, the user holds the back wheel off the ground and cycles through the gears with the shift knob. The derailleur obliges, clicking smoothly through its full range, no issues there.
A series of taps of the helmet with a ¾ finger gloved hand is warranted, both to shake off some dust from the goggles, and to praise the helmet for its protective powers. Readjusting the extruded aluminum seat post, which has shifted height and angle during the crash, the rider clicks back into the pedals, and rolls off down the beautiful green hills of the Chilean Andes.
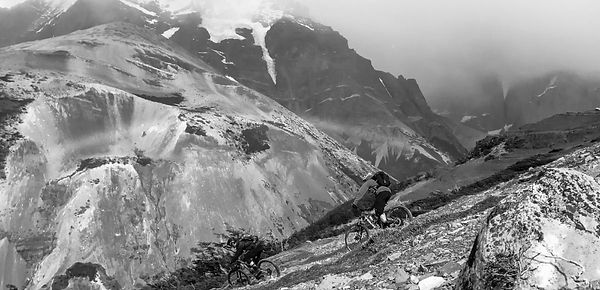
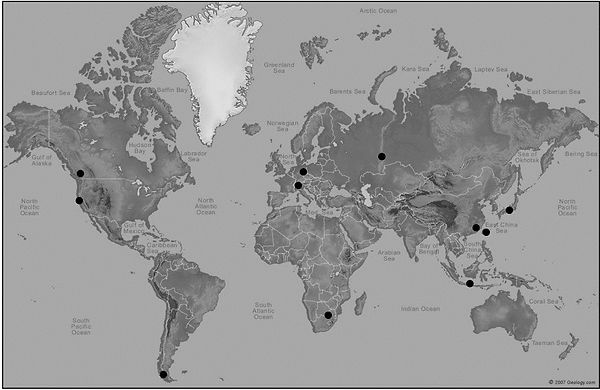